[Updated/corrected, April 22, 2015] The world’s most powerful particle accelerator — the Large Hadron Collider (LHC) — is scheduled to restart in March of 2015, after a two year hiatus for repairs and upgrades (and data analysis). The machine is located at CERN (in Switzerland) the largest and most prestigious high energy physics laboratory in the world.
The power of such “atom smashers” — actually, in the case of the LHC, they’re protons — is gauged in terms of electron volts (eV) which is the energy used to accelerate the groups of protons before they smash into each other and shattering into (hopefully) other constituent particles. Before the LHC came along, the most powerful accelerator was the Tevatron at Fermilab (a.k.a. Fermi National Accelerator Laboratory near Chicago, IL) which could collide the protons and anti-protons at a maximum (potential) energy of two TeV (trillion elctron volts); the few other colliders were capable of only billions of electron volts (GeV), at most.
The mighty LHC has a maximum power of 14 TeV (trillion electron volts), but will start out at a more modest 6.5 TeV and then, over the course of the next two years or so, it will rev up to “perhaps 12 TeV” according to James Gillies, senior scientist and program head at CERN, and one of the five experimental physicists at this past Friday’s symposium panel entitled ‘What Can We Expect from the Second Run of the LHC in 2015?’ [note: the panel discussion was part of the annual AAAS/Science Meeting in San Jose, CA]. A collision energy of 12 TeV — should it be achieved — will be the highest particle collision energy ever attained but still shy of the machine’s maximum power (perhaps the thought is it’s best to keep a little power in reserve). And with this increase in power comes an increase in the rate of particle collisions from 360 million per second to 700 million per second, providing nearly double the amount of collision data.
The prime mission of phase II of the LHC is to determine why the cosmos “prefers” matter over anti-matter (and, therefore what happened to all the antimatter?).
The remaining four panelists were: Frederick Bordry (of CERN), Beate Heinemann (University of California, Berkeley), Michael Williams (MIT) and Peter Jacobs (also of CERN). All five panelist refer to themselves as experimental physicists (those who conduct actual experiments) to distinguish themselves from the purely theoretical kind. There exists in the physics community a generally good-humored rivalry between the two camps. And, after all, theory and experimentation are two sides of the same effort: to understand the fundamental nature of the physical universe.
A Brief History of the LHC
Readers may recall that the LHC was scheduled to go on-line back in 2007, but due to a series of delays and repairs (most involving faults in the machine’s magnetic couples), it didn’t actually become operational until 2009. However, the LHC didn’t start taking data until March of 2010. Over the course of three years, the LHC was put through its paces to generate most of the known particles in what’s known as the Standard Model of Quantum of Physics — save for one: the fabled Higgs boson* (what one physicist originally called the “god particle” — because it had the power to give mass to other particles — and the name just stuck, even though most physicists don’t care for the term).
In the flurry of sensational news stories prior to its start up, some of the public grew alarmed; the machine was the first machine ever built that was “capable of destroying itself” (What if it took the rest of us with it?). And then, after a visit from the distinguished theoretical physicist and Nobel Laureate Stephen Hawking, during which he remarked (with the press present) that he was not interested in the Higgs, but in creating a “mini black hole”….Well, that news traveled at near light speed around the globe, and suddenly, CERN physicists were fielding incessant anxious questions and doing damage control (in the interest of good public relations). Eventually, the hullabaloo dissipated and the LHC finally started smashing protons via its two opposite-conducting, 17 mile / 27 km long, underground tunnels, as it was designed to do.
Then, in 2012, near the very end of its scheduled three year run — and clocking in at a “mere” 125 – 127 GeV — the LHC’s two main detectors (ATLAS and CMS) started detecting a consistent signal in the LHC’s collision data with a “3 Sigma” rating or better (that’s physicist jargon for strong confidence; “5 sigma” being a sufficiently high confidence rating to declare a true “discovery”). It took some time before the data could be confirmed and CERN scientists tried their darnedest to keep their excitement contained for as long as possible, but ultimately, it was officially confirmed (on 4 July 2012): the international LHC consortium of physicists had indeed found the Higgs (or a particle just like it). And there it was: the once theoretical Higgs was now experimentally validated — a phenomenon of science that always amazed Einstein — and the Standard Model was “complete”.
*In actuality, the Higgs boson was/is a theoretical construct that allows physicist to probe the Higgs field, which has a non zero constant value and thus cannot be “turned off” like the electromagnetic field. Partly for this reason, the Higgs boson “gives” mass to many other particles (even to some that should not have mass according to symmetry theories). There may in fact be more than one form of Higgs boson, and many problems and puzzles surrounding this Higgs boson remain, such as the “logarithmic conversion of the mass of the Higgs” (which, according to physicist Michael Williams involves a puzzling and transient conversion of the Higgs to a top quark and anti-top quark] ; see also: The Higgs Hierarchy problem)
Joy and Despair (and some Hope) in Physics Land – Are Super Symmetry and String Theory ‘Dead’ Theories?
As momentous as this discovery was for physics, not everyone was entirely happy; many were hoping to find some more exotic particles whose existences might extend or expand the experimental horizons of the discipline, or perhaps even redefine the Standard Model itself. One specie of particle that did not appear (at the energies achieved and expected for some of them) — superpartner particles — was one such disappointment, and so, the beautiful theories that depended on them being found — like String Theory and Super Symmetry (or SUSY for short) — seemed to be DOA.
Ah, but beautiful and elegant theories die hard in physics.
In response to a two part question by this author (“Is SUSY dead? And what about String Theory?”), panel member/physicist Beate Heinneman replied: “No, SUSY is not dead…and neither is String theory…super symmetry predicts new particles which are related to the standard model particles…SUSY actually solves three problems we currently have in this would that we don’t understand and all of them are related to the energy scale of the energy probes…So, we think that it is very likely that these particles [will] show up…However, if they don’t…the theory would not be dead…There is something like elegance which people used to guide their thoughts about Nature…So one can solve each problem; one can find one way to solve problem A, another for problem B…But it is much more elegant to have one theory that solves problems A , B and C…So, the more we see what it does [Supersymmetry] in its natural form…We see that it does have some theoretical advantages compared to some others…So, in the second run we can look much better for Supersymmetry…it is a real possibility.”
James Gillies then added his view of things: “The standard model is constructed around symmetry groups (other symmetry theories)…maybe there’s another symmetry group, and so, we will look for that…The Higgs particle may be several particles…we speak of looking for additional particles…regardless of SUSY or String Theory…Extra dimensions of space and time is another possibility…These things don’t get quite as much press, but there’s a large bag of things that we’re looking for besides Supersymmetry and String Theory.”
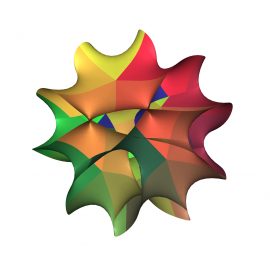
Peter Jacobs joined in with this response (to my questions): “I think I was the only person to mention String Theory in my talk…but being an experimentalist, not a theorist…String theory is a wonderful mathematical construct in terms of a beautiful solution in search of a problem…and some people think that this quark-gluon plasma theory that I spoke of is a point of contact with the calculations in String theory…the connection is not established…but it seems that the kind of things we measure maybe able to do analogous calculations…String Theory is not like QCD…It is possible that the kind of physics I described may be a point of contact, but this is far from established. But it’s fun to think about, and fun to debate…When I look at things strictly in terms of the calculations, the first thing I think is: is this a profound calculation or is it somebody’s small model and what if we disprove it? Have we really said something profound about theoretical physics?…This is a very complex question that we are are still trying to grapple with…there may be a common discussion to be had…but I ‘m not really yet ready to connect experiment with theory.”
Finally, Michael Williams commented: “As far as SUSY…We know that it is a broken symmetry…It’s particles are not the same masses (that we are dealing with now)…Whatever the mechanism is that breaks it…we don’t know what the masses will be…we have no idea what that will be…So, we don’t really know what the masses should be, or the problems that it solves…We’re probably two orders of magnitude away from getting to masses that totally solve the problem…that’s the reason why you’re not going to kill SUSY…we might find it [the missing mass]…but we’re not going to kill it.”
More Q & A : Plans and Possibilities
At the start of the Q & A, the panel moderator, James Gillies, asked: “The US has contributed about 500 million for both the accelerator and the experiments (note: this was mostly for the ATLAS detector primarily) we’re send a few hundred million more (for this second phase)…Americans are busy filling out their 2014 tax forms…Are they getting their money’s worth?”
To which French physicist Frederick Bordry replied: “I think definitely it is a very good deal…the machine itself is five billions of dollars…the US contribution was less than 200 million (note: Bordry seemed here to disagree with the cited dollar figure of the US contribution to the LHC)…We are looking at half a billion in operations per year (to run it)…with no contribution (yet) from the US…and the biggest number of researchers is coming from the US…So, I think that is a very good [deal].”
As far as when the consortium will power up the LHC, and at what energies, Bordry added:
“The strategy was to start smashing as quick as possible. We could start smashing without any quenching [note: this refers to the unexpected loss of superconductivity of the magnets the machine uses to accelerate the proton beams]…I think we should be ambitious…We expect to do that (rev up to 6.5 TeV) in one to two months…to find out about these quenches if they are due to the beam…and find out about the magnets loosing their memory…and, if we want to increase it [the energy of the beam], how much do we increase it?”
Other questions and answers:
Regarding finding [evidence of] Dark Matter via missing mass and momentum…If a new particle is found, how will you tie that to dark matter?
(Beate Heinnemann) “That is s good question…so we can not do that directly…we can only observe that there is some particle that we don’t detect that is missing…then we understand what particle is produced together with the dark matter and using theory we can understand the rate and whether it is consistent with some theory that contains dark matter. But, to really see the dark matter…it won’t really solve the dark matter mystery, what we really want to do is conduct (underground) experiments to detect particles that are left over from the Big Bang, and so, if you find it’s a good suspect then…it may well be dark matter but…it’s a bit like: if it smells like a cat and meows like a cat it probably is a cat…even if you don’t see that it’s a cat.”
So, will it be a new particle?
(Heinemann) “Maybe we can say that what the headline would be : We have found something that shows the Standard Model is incomplete…We find some disagreement with the data with the standard model…then to actually figure out what exactly that is….what particle or force it is…there are several ways you could get experimental signatures…but that would take awhile to work out…So the headline would just be, must be: we found something new that we never expected…and that would be the fun really, to figure it out…and there a lot of theoretical physicist who would help us figure this…what this particle is…Does it have any brothers or sisters? What is the complete theory? So, this could take decades.”
SPECIAL THANKS TO Youhei Morita for his technical corrections and suggestions for this updated article.
Top image: Simulated Large Hadron Collider CMS particle detector data depicting a Higgs boson produced by colliding protons decaying into hadron jets and electrons (image: CERN; CC BY-SA 3.0}
Bottom (second) Image: A 2D slice of the 6D Calabi-Yau quintic manifold. (Uploaded by Ronhjones; CC BY-SA 3.0)
hi